UNEXPECTED DISCOVERY
ECU medical research team discovers paradigm-shattering muscle phenotype
Researchers at East Carolina University have discovered that a muscle in the foot appears fundamentally different from other muscles because of how it behaves without oxygen. Their inquiry is just beginning to try and figure out why.
Skeletal muscles are used for breathing, movement, temperature regulation — the activities of life that blow through oxygen because of its necessity for maintaining energetic potential, said Espen Spangenburg, professor of physiology and chair of the Department of Anatomy and Cell Biology at the Brody School of Medicine.
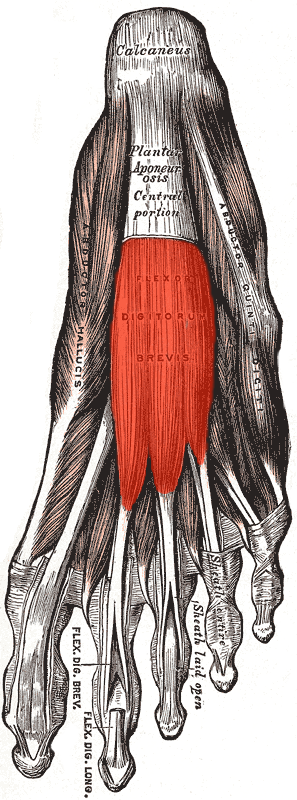
The red highlighted section of the foot represents the location of the flexor digitorum brevis muscle in the human foot. (Photo courtesy of Wikimedia Commons)
The flexor digitorum brevis, FDB for short, is a skeletal muscle located in the feet of mammals. The FDB is commonly used in biological experiments because the muscle is amenable to many different types of physiological measures.
Spangenburg primarily studies skeletal muscles, but several years ago he pitched in to help fellow Brody researcher, Joseph McClung, with a study looking into therapies for peripheral arterial disease. The study called for the reduction of blood flow to the extremities of subjects in pre-clinical models and because the FDB is the muscle furthest from the heart, it should have been the first to show signs of damage from the blood flow restriction.
But that didn’t happen.
“One of the people in my lab came in and threw his stuff in my office. He was really upset,” Spangenburg said. “He goes, ‘I can’t make this muscle die’ and he was really upset about it. I kept looking at him and thought, ‘Wait, we’re going about this all wrong.’”
If the muscle doesn’t die by limiting the flow of blood, and in turn oxygen, Spangenburg thought, then maybe there is something odd happening. He needed to shift his team’s focus to figure out why the muscle is surviving in conditions that current science says shouldn’t be possible.
The FDB samples that Spangenburg and his team studied weren’t immortal like a movie superhero. Any number of insults caused the muscle tissue to fall apart, but for some reason the FDB was able to survive much longer without oxygen than other types of skeletal muscles.
Oxygen is necessary for skeletal muscle function because it works within the mitochondria to produce ATP, a molecule that is necessary for cell processes like muscle contraction and nerve communication. If the oxygen deprived FDB muscle didn’t need oxygen to meet its energetic demand, then Spangenburg’s team wanted to know what other mechanisms could provide the ATP.
Glycolysis, breaking down of carbohydrates stored in muscle tissue, seemed like a viable option, and their work found that it could account for some of energy that was keeping the FDB tissue viable, but testing showed that glycolysis wasn’t enough by itself.
Hypotheses
Why does the FDB muscle seem to behave differently than other muscles?
Spangenburg was stumped, and he took his team back to the drawing board. He reached out to another ECU researcher, Kelsey Fisher-Wellman, who specializes in the relationship between mitochondria and cancer. The two labs pooled their resources and began what Spangenburg calls a “discovery-based approach” by screening thousands of proteins and comparing them against other muscle profiles. They identified a number of proteins that were unique to the FDB and show promise in explaining the differences between the FDB and other skeletal muscles.
“We thought we’d see a lot of energy-based things and that’s not what we’re really seeing. It seems to us that the muscle has some way of controlling its environment,” Spangenburg said. “One of the things that we thought it might be doing is hibernating. We still haven’t disproven that yet.”
Fisher-Wellman said the discovery that Spangenburg’s lab made is fundamental, meaning the potential clues about mammalian biology unlocked from the findings could have wide-ranging impacts that have yet to be considered.
“There is something fundamentally different about this muscle compared to others,” Fisher-Wellman said. “That’s the exciting part. Once you fill in those gaps, then it becomes a little bit easier to see where the findings can be applied.”
Fisher-Wellman’s lab at ECU had a role in the discovery. His team ran the proteomics necessary to validate the differences between the FDB and other muscle tissue used as the control for the experiment. Proteomics is a technique that involves reducing the structure of tissue, basically grinding it up, which allows for analysis by a mass spectrometer, a high-tech machine that can suss out the molecular components of the tissue being studied.
Fisher-Wellman’s lab conducted the proteomics procedures on FDB muscle samples as well as two other types of muscle tissues from the same donor to use as controls. Having two controls, Fisher-Wellman said, solidified the validity of the findings.
“I’m just as surprised by the data as everyone else. When I first heard about it, I was like, ‘Come on, that can’t be real’, and then you see the data over and over and over … that’s wild,” Fisher-Wellman said. “I’ll be interested to see where they go with it. Someone’s going to be busy for a while.”
One of the persistent challenges in studying muscle tissue is plasticity — the ability for the muscle tissue to adapt to the requirements placed upon it. A runner can switch from sprinting to long distances and each style of movement demands the same muscle to behave differently. If an astronaut spends long stretches of time in space under zero gravity, the muscle changes to meet the metabolic and locomotive needs of the individual. Because of muscle tissue’s inherent plasticity, isolating variables becomes even more of a challenge for researchers.
Another perplexing aspect of the finding is why a muscle necessary for movement, but not fundamental for life support — think heart or diaphragm muscles that keep your heart beating and lungs breathing — would have adapted to survive for much longer than other muscle types without oxygen. While Spangenburg has some ideas, perhaps the heavy and repetitive stressors placed on the foot for movement, he is still not confident in proposing a hypothesis.
The lack of an obvious reason why the FDB behaves as it does is equal parts thrilling and frustrating.
“I would stare at the ceiling at three in the morning, scratching my head. I was determined we were going to figure this out,” Spangenburg said. “It was a reminder of how little we understand. Sometimes we think that we have things figured out, and then you get a moment that blows our complete understanding of things out of the water.”
Everett Minchew, a Ph.D. candidate who works in Spangenburg’s lab and will graduate in December, is encouraged by the fundamental nature of the findings, which may have broad practical application across medical research disciplines. Although basic science isn’t always basic, he said the results are relatively easy to translate to non-scientific audiences.
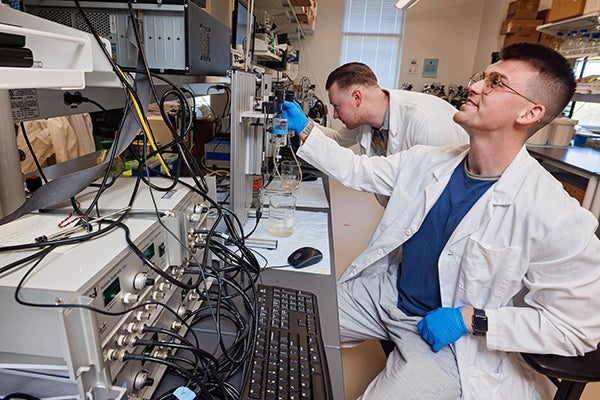
Doctoral candidates Everett Minchew, right, and Nick Williamson work in the Spangenburg Lab at East Carolina University. (ECU photo by Cliff Hollis)
DOCTORAL DISCOVERIES
Everett Minchew comes from a medical family in New Jersey. After job shadowing a few doctors it became evident that physiology was his calling and he wanted to dive deeper into how the body works.
Minchew believes the physiology research team at ECU is on par with any marquee institution in the country, and he is proud of being a part of Spangenburg’s lab.
“You see big discoveries out of the big-name schools all the time; to have a little sliver of that that here at ECU is pretty exciting,” Minchew said.
“The beauty is that when my friends and family ask me about my research, I can explain the project in layman’s terms and they get just as excited,” Minchew said. “It would be a totally different story if I was working on something like a very specific protein structure that isn’t easily understandable.”
The math problem
The doldrums of science might be the numbers. Ah-ah moments are few and far between.
“You don’t ever want to hear people call your publications incremental. We’re in a weird environment now where everybody wants the complete answer now. We started collecting data and then started reproducing, then we were sure we were on to something. I was like, ‘Well, I’m not going hear incremental with this,’” Spangenburg remembered.
First, he had the get the math worked out.
Spangenburg was at home one day working to unknot some numbers. His son, Quincy, who is a junior at D.H. Conley High School in Greenville and in a college calculus course, asked his father what he was working on.
“I happened to be working from home that day and I was bent out of shape because I knew I should be able to mathematically calculate it and I couldn’t figure it out,” Spangenburg said. “I pulled him over and said, ‘Here’s my situation.’”
Quincy said that data looked like polynomial function and he thought he could use a tangent line to calculate the answer Espen was trying to derive from the data. Espen gave Quincy the data and an hour and a half later Quincy returned and told his father that he had it figured out.
“We had to make some adjustments to his approach, but he literally developed a method for how we would assess the data,” Spangenburg said. “But then I didn’t know what to do, because typically when somebody adds to the research like that, you would add them to the paper.”
Spangenburg was in a quandary — how would it reflect on both him and his son to add Quincy to the paper as a co-author? Would it be seen as nepotism? Was it ethical?
“I called up some of my colleagues and said, ‘OK, this is what happened.’ What do I do? Do I add him to the paper? This is weird. I don’t want this to look weird,” Spangenburg said. “And they all came away with the same thing — ‘Could you have done it?’”
Not the way Quincy did it, in a manner that was tangible for the readers, Espen responded. Quincy is an integral part of the research, his colleagues responded — you have to add him as a co-author.
Regardless of whose name is on the paper, the team’s findings are already causing researchers at other institutions to question assumptions about how the body works.
“I have one guy who just sent me a whole experiment with the FDB muscle and asked, ‘Am I wasting my time? Like should I even be doing this?’” Spangenburg said. “This finding could change what we put in textbooks down the road because this muscle is an exception to the rule. And that means that there might be other exceptions. It may not be the only one.”
Spangenburg’s eyes light up when he speaks about the possibilities from his team’s findings.
“You start to realize how complex the systems are. Sometimes we underappreciate the complexity.”